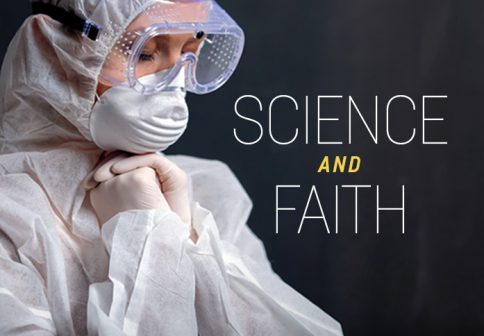
An honest approach to the philosophy of science and/or religion needs to admit to human limitations.
Leonard Brand and Arthur Chadwick
Have you ever seen an animal peel a tiny wrinkled fruit such as a raisin?1 Chipmunks do. I (Brand) have watched numerous chipmunks sit and manipulate a raisin in their paws. When they run away, there is a little pile of raisin peelings where they were sitting. Chipmunks are such adorable creatures to study, but what is the difference between observing the cute things they do and scientific study? To qualify as science, the observations or experiments must be done in a systematic, planned way so other scientists can verify the study by repeating the experiments or observations to see if the results come out the same. Random observations will not do.
Scientific study is designed to answer a question or to test a hypothesis. The research may answer the question or only lead to other questions to be studied. The idea to be studied could come from anywhere, but it must be tested by the scientific process. Science may be defined as a systematic search for truth through experimentation or observation. It is a powerful method for discovery, but there are some limitations. Some ideas or events cannot be studied by science (like the miracles of Jesus or which automobile design is more esthetic).
The Impact of Darwinism
Charles Darwin’s theory of evolution has been very successful as a good scientific theory. A few decades ago, an article was published titled “Nothing in Biology Makes Sense Except in the Light of Evolution.”2 That article illustrates the scientific community’s confidence in the theory of evolution and the extent to which it has been successful in organizing and explaining a broad range of biological data.
Chipmunks provide an example of this success. Only one species lives in the eastern half of the United States, but the western states have 21 species of chipmunks. Why are there so many species in the West but only one in the East? The evolution theory provides one possible answer.
The western United States has a great variety of habitats suitable for chipmunks, including dense brush, semidesert pinyon pine forests, yellow pine forests, and high-altitude lodgepole pine forests. Many unsuitable habitats such as deserts or grassy plains separated small populations of chipmunks in isolated geographic pockets. As each population became adapted to its habitat, some populations became different species through natural selection. However, in the eastern United States, the original forest environment was relatively uniform, and few natural barriers were adequate to isolate small populations of chipmunks and thus to produce new species. Evolution not only provides explanations such as these but also suggests experiments to test these explanations. In many cases, the theory successfully predicts the outcome of the experiments, giving scientists great confidence in evolution.
For nearly 1,900 years, most of the Christian world accepted without question the creation account in the Book of Genesis. Then, in a few decades, Charles Darwin and his colleagues changed all that. For many people today, evolution is the only valid account for the origin of all living things. Why did Darwin’s theory have such an impact? Has it made the Christian’s belief in a Master Designer untenable, or have some factors been overlooked?
The history of science shows that even very successful theories sometimes need improvement or replacement. Therefore, it is appropriate to continue examining the foundations of evolution theory and to ask hard questions. Are all parts of the theory equally well-supported? Has some important evidence been overlooked or underestimated? Do aspects of our logic need to be cleaned up?
Such probing benefits both science and religion if appropriately conducted. We must be honest with the uncertainties in the data and be careful to distinguish between data and interpretation. An approach to these and similar questions must affirm the integrity of the scientific process while maintaining a context of faith. The task must be approached with humility and open-mindedness and a recognition of when the data point to dimensions of reality beyond our current understanding.
The success of science has encouraged a tendency to believe whatever science claims. An understanding of both the strengths and the limits of science can enable us to relate to it more realistically.
A Definition of Science
Science can be defined as a search for truth through repeated experimentation and observation. We can recognize two parts of that endeavor: First is the content of science—the things that science has learned and the system of organizing that knowledge. Certainly, this is an important part of science. But if we stop here, we miss the most exciting and valuable part—the process of searching for and discovering something new.
The Scientific Process: The Search
Scientists, in the process of discovery, formulate hypotheses or theories, collect data, conduct experiments to test theories, and develop generalizations called scientific laws. This scientific search process has two primary parts: (1) the collection of data and (2) the interpretation of data.
The activities of a scientist can all be clustered into these two categories. Collecting data is an absolutely essential step in science. It can be exciting, or it also can be quite routine. Then determining what the data mean is the most rewarding and creative aspect of research—the realm of ideas and the application of those ideas to make sense of the data and formulate a plan to continue the search through further data collection.
Science is quite freewheeling, and different researchers approach data collection and interpretation in different ways. Working in labs and field-research sites, people learn to do science from experienced scientists.
When the scientist has an idea, it is expressed as a question that can be addressed with the scientific method. For example, while watching squirrels, we hear them make a sound. Are the squirrels communicating? And if so, what are they communicating to other squirrels? If we are in the desert and see several types of rock formations containing fossils, we may ask how those rocks and fossils originated. What is the process by which they got there? After posing questions, we try to determine what kinds of data are needed to answer them. It is often necessary to break a question down into more specific questions.
What type of data could answer the question about the squirrel calls? It would help if we at least knew under what circumstances those calls were given. For example, is the call given when a predator is approaching or when a neighboring squirrel comes close to the caller’s food cache? As we observe the squirrels, we write down everything about the circumstances. These data could begin to answer our questions. The rocks and fossils are a little harder. We cannot watch them form, but we can observe what is going on today when rivers and streams and ocean currents deposit sand, mud, or other sediments. We can compare these modern processes (modern analogues) with the characteristics of the rocks to see which deposit is most similar to the rocks we are studying.
Imagine that we are archaeologists who have discovered several broken pieces of glass from an ancient ruin. If we are honest, we cannot argue with descriptive data like these—the shape or composition of the objects. These facts are objective data—features that can be weighed, measured, and defined by anyone with the same results. But no matter how accurately we weigh and measure, the data are still just broken pieces of glass. The research is incomplete until we can make sense of the data through interpretation.
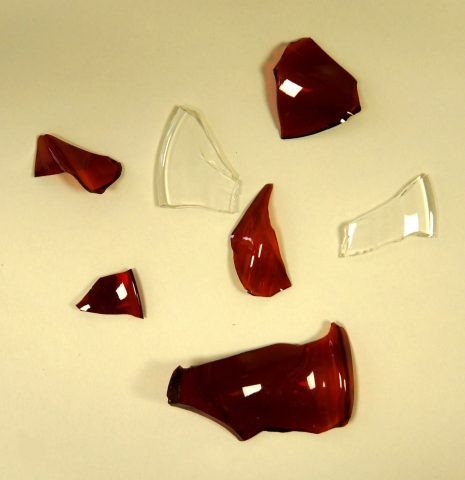
Interpretation involves examining relationships among pieces of data. In this case, the relationships need to be expressed in terms of what an object was like and how it was used. We probably could not answer these questions directly from the data since they tell us only what the broken pieces of glass are like. We have to relate them to what we already know about similar objects and archaeological theories. Then we can devise a hypothesis about what the original glass object was like. Interpretation is not an objective process. We must use creativity and imagination, but we cannot let them run wild. The data create boundaries for our hypotheses—the color patterns should make sense, and the curvatures of the reconstruction must fit the shape of the glass pieces. The possibility of objectivity is reduced by a couple of other factors. In science, data are often not as objective as the shape of pieces of glass, and how we describe data and even what data we collect are usually influenced by our theories.
Is our hypothesis correct? How would we know? A lot of data is missing, so we can’t be sure. As often happens in science, another scientist may look at our interpretation of the data and decide that it was not done correctly, so he or she develops another hypothesis. Broken vases, like jigsaw puzzles, likely go together in only one way. However, if the majority of the pieces are missing, we can probably arrange the remaining ones in several different ways that look logical. For this reason, there can be differences of opinion about our glass object. In science, especially in fast-moving fields, interesting dialogue is common among people who have different interpretations. But what we ultimately want to know is which hypothesis is more nearly correct. How do we determine this? The only way is to search for more data.
Perhaps we are successful in our search and find two more pieces of glass. One has a ridge on each end, which fits with the second hypothesis. But the other piece, with a flared top, does not fit either hypothesis. So, we develop a new hypothesis that fits all the current data. Now is it correct? This is still not certain, since we don’t even know how much data are missing. In this case, we are going to cheat and look at the original. Part of our hypothesis was about right, but other features were still incorrect.
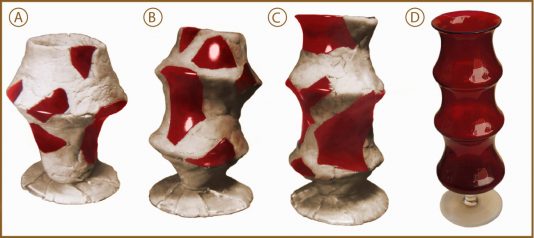
The glass vase illustrates the self-correcting aspect of science: As we gather more data, we improve our chances of eliminating incorrect ideas. The accumulation of data shows where problems in our hypotheses or theories still lie and helps us think of better hypotheses. Of course, complications appear along the way. What if the glass pieces are actually part of something quite unrelated to a vase, but we are considering only hypotheses about vases? Our theories strongly influence our interpretations of data, and wrong theories can slow down the scientific process. In that case, an improved understanding of nature may depend on new, creative interpretations of existing data or may await the discovery of additional data that clarify our thinking.
Factors That Make a Theory Useful to Science
In the 1790s, a priest named Spallanzani did some fascinating experiments with bats. He covered the eyes of some bats and the ears of others. From the results, he reached the conclusion that bats have to use their ears, not their eyes, to navigate successfully at night. Spallanzani could not hear the ultrasonic sounds the bats were using. Consequently, his conclusions did not make much sense with the knowledge available at that time. But the data still supported his conclusion.
The prominent scientist Cuvier responded by proposing a theory that bats use a highly specialized sense of touch to find their way around in the dark. He had no evidence for his theory, but he was more prestigious in scientific circles, and more scientists accepted his explanation.
More than one hundred years later, Spallanzani was vindicated when the discovery of echolocation made sense of his observations. Bats give out ultrasonic cries, listen to the echoes, and use them to find their way around.
A good scientific theory or hypothesis has several specific characteristics. The first one is illustrated by Spallanzani and his bats:
1. A theory organizes and explains previously isolated facts. When a new field of inquiry is just beginning, there may be a lot of facts. But it is hard to see how they relate to one another (like the bats and their use of their ears for navigation), and people may have different ideas on how to put them together. A successful theory makes sense of these previously unrelated facts. This happened with the new research one hundred years after Spallanzani.
2. A good theory also suggests new experiments and stimulates scientific progress. Donald Griffin’s theory of echolocation suggested experiments to test that theory. Experiments are not selected randomly. They are generally chosen because some theory suggests they will yield new insights. Experiments are done to test a theory.
3. A theory should be testable. We should be able to think of data that may potentially falsify the theory. If it is not possible to do that, then the theory may not be very useful. Echolocation could be tested by experiments, and the theory passed the test.
To further illustrate what is meant by a testable hypothesis, compare these three hypotheses:
A. Ants behave the same way in undisturbed underground tunnels as in glass observation chambers.
B. Goldfish need oxygen to live.
C. Extrasensory perception exists.
Which of these three can be tested? How would you prove or disprove the first hypothesis? Probably there is no way to find out what ants do many feet underground without seriously disturbing them. If we disturb them, we cannot possibly determine what they are doing in undisturbed tunnels. If we really want to understand ant behavior, we have to know the answer to this question, yet it is unanswerable. Science often has to live with such uncertainties.
Is the second hypothesis testable? A suitable experiment would be to seal up the goldfish bowl and see what happens, or provide the fish with an artificial atmosphere that does not contain oxygen. If the fish survive the experiment, then the hypothesis has been disproved.
Can the third hypothesis be tested? One can design an experiment in which a man who claims to have powers of extrasensory perception is asked questions about thoughts or events in another place. If he can answer the questions correctly, it would indicate that something unusual is happening, but we still would have to determine exactly what it was. However, if he could not answer the questions, he might still claim that he usually can do so but was unable to because the scientist was watching him. If that happens, what are we going to say? The experiment could not disprove the concept.
Keep in mind that the discussion above is describing the ideal situation, but science often goes beyond ideal, testable phenomena. Theories about the distant universe or about events in Earth history may not be genuinely testable because we were not there and cannot get there, but they still may constitute legitimate science. Science is not always as objective and straightforward as we might wish.
4. In experimental science—such as chemistry, physics, or physiology—experiments done to test a theory or hypothesis should be repeatable. An experiment should be defined in precise, quantitative terms so that somebody else in another lab can do the same experiment and get the same result. Do fish in different research labs respond in the same way to a lack of oxygen? In contrast, hypotheses about subjective concepts like human opinions or attitudes are very difficult to test with repeatable experiments.
5. The last characteristic of a useful theory is that it predicts the outcome of experiments that have not been tried yet. There is a reason this is important. If we do an experiment to test a theory and then, after finishing the experiment, we try to show that the results support our theory, that is not convincing. But now let’s go back to the 1790s. Spallanzani’s work suggested that if we could hear all that a bat hears, we would hear the sounds that a bat uses for navigation. More than a century later, that implied prediction was tested, and Spallanzani was right. The fact that the ultimate result was predicted in advance gave great credibility to the theory of echolocation.
These five characteristics of a useful theory do not say that the theory has to be true. Is that disappointing? We hope our theory is true, but how would we know? That is what we are seeking to discover with our experiments. We don’t know ahead of time whether a theory is true. We must wait for the results to come in, and often that can take a long time. A theory can be wrong and still lead to significant scientific advancement before we find out it is wrong.
The Source of an Idea Is Not What Determines Its Value
How do we get the ideas that we formulate as hypotheses? The scientist Archimedes had an interesting experience as the result of a task given to him by his king. The king had been given a crown by some of his subjects. They told him it was pure gold, and the king asked Archimedes to determine whether that was true. Archimedes was thinking about this, the story goes, when he went to the public bath. His alert mind noticed that when he got into the tub, the water raised along the side. An idea occurred to him: perhaps an object submersed water displaces a volume of water equal to the volume of the object. He was so excited, he forgot his clothes and ran down the street yelling, “Eureka [I found it]!”
Part of the story may be apocryphal, but apparently Archimedes did get information from his bath observations that helped him accomplish his task. By putting the crown in water, he could determine its volume. Then he could weigh it and calculate its density, which was not the density of gold. Someone probably lost his or her head over that, but it wasn’t Archimedes. Archimedes’ experience illustrates how chance observations sometimes lead to an idea. Of course, it was important that Archimedes’ mind was prepared to recognize the significance of his chance observation.
Another example comes from research done on white-footed mice (genus Peromyscus). We (Brand and team) needed to catch mice on the dry, barren islands in the Gulf of California. We set traps in the valleys in a typical habitat and caught only two or three mice per one hundred traps, as would be expected. While walking along the beach looking for rattlesnakes and fish-eating bats, we saw something scurry over a rock. Out of curiosity, we started turning rocks over and found a Peromyscus. We “knew” that Peromyscus do not live on beaches. But when we set traps along the beach, we caught 30 mice instead of the expected three. The mice on these islands had moved into a unique habitat, apparently making use of the food supply washing in from the ocean. A chance observation of a mouse darting over a rock led to a discovery we would never have made otherwise.
Previous experience or known theories are also important in suggesting ideas. It is known, for instance, that if two birds are in conflict over a territory, the bird defending its own territory has a psychological advantage and nearly always defeats the intruder. A friend and I (Brand) wondered if chipmunks might show the same behavior. Our research revealed that chipmunks are not as territorial as birds, but they do become more aggressive when close to their nests. Known concepts about birds helped us devise testable hypotheses on chipmunk behavior.
Scientists sometimes say that an idea came to them in a dream or just occurred to them. This seems very unpredictable. Science can function that way because ideas can come from all kinds of places in all kinds of ways. Where an idea comes from cannot be defined in objective terms, so what does that do to science? The characteristics of a scientifically useful theory are helpful here. Can the theory be tested? That is the answer. Archimedes’ bathtub observation could be tested by experiments. Where an idea originates is irrelevant. If we can do experiments to test it, any idea can be scientifically useful.
Picture a scientist visiting a primitive culture and observing a witch doctor treating patients with magical herbal cures. She hopes to find some plants with medicinal value. Is that an acceptable source for scientific ideas, or must those ideas arise through the normal scientific process? Since she can test the plants to see if they really are medicinal, it does not matter where the idea comes from. Could productive research ideas even come from the Bible?
Data Do Not Automatically Lead Scientists to Truth
Some influential people, like English philosopher Francis Bacon, promoted the idea that data faithfully lead us to truth. However, Bacon was overly optimistic. Data almost never directly suggest the interpretation, and data do not guarantee that interpretation of the data will be correct. The scientist must relate the data to theories and “known facts,” working creatively to interpret them. In our study of the broken glass pieces, we had to relate those data to information we already knew in order to develop an interpretation. Of course, some of the theories and known facts—and thus the interpretation—might be wrong. Scientific explanations develop through time as we interpret data, evaluate our conclusions, and learn from our mistakes.
When the data do not all seem to point to the same conclusion, scientists generally choose the conclusion they think is supported by the greatest weight of evidence. But we always have only part of the potential data, and our own preferred theory can influence our evaluation of the data. When Galileo Galilei was arguing that the Earth rotates around the Sun, there was some important evidence that seemed to say Galileo was wrong. The apparent weight of evidence at a given time may actually point in a wrong direction. The best we can do is to trust that continued research will reveal such mistakes in time. Of course, in evaluating the weight of evidence, some sources of information may be considered much more reliable than others. Scientists will always trust research journals more than newspapers. Informed interventionists (creationists) will take the Bible account of origins more seriously than other scientists will.
Does Some Truth Exist Outside of Science?
There must be true answers to our questions about nature, and our theories and scientific models are tools that assist us in the search for those ultimate truths. Yet an idea that is true in an ultimate sense may not be scientifically useful. To say that something is “not scientific” could mean two very different things. It could mean that the idea is false, or it could mean that science cannot determine whether it is true because it cannot be tested. If we were able to see from God’s perspective, we could define a portion of human ideas as true and recognize others as false. We would find that science is able to test some of these true ideas and to convince us of their truthfulness (e.g., gravity). Science can also test some of the false ideas and show that they are false (the Sun rotating around the Earth). Other ideas in both the “true” and “false” categories cannot be tested scientifically.
There are things in religion that are not amenable to scientific investigation. Science cannot test them. This doesn’t mean they are false. They just may be outside the realm of what science can deal with. Did Jesus actually heal people? Science cannot answer this question. Many other questions similarly cannot be answered. An honest approach to the philosophy of science and/or religion needs to admit these human limitations.
Leonard Brand, PhD, is Professor of Biology and Paleontology and former Chair of the Department of Earth and Biological Sciences at Loma Linda University in Loma Linda, California, U.S.A.
Arthur Chadwick, PhD, is Professor of Geology and Biology at Southwestern Adventist University in Keene, Texas, U.S.A.
NOTES AND REFERENCES
1. This article is adapted with permission from a chapter in Leonard Brand and Arthur Chadwick's Faith, Reason, & Earth History: A Paradigm of Earth and Biological Origins by Intelligent Design (Berrien Springs, Mich.: Andrews University Press, 2016).
2. T. Dobzhansky, “Nothing in Biology Makes Sense Except in the Light of Evolution,” The American Biology Teacher 35:3 (1973): 125–129.
1. This article is adapted with permission from a chapter in Leonard Brand and Arthur Chadwick's Faith, Reason, & Earth History: A Paradigm of Earth and Biological Origins by Intelligent Design (Berrien Springs, Mich.: Andrews University Press, 2016).
2. T. Dobzhansky, “Nothing in Biology Makes Sense Except in the Light of Evolution,” The American Biology Teacher 35:3 (1973): 125–129.